Water Gaia: The Earth as Aquifer
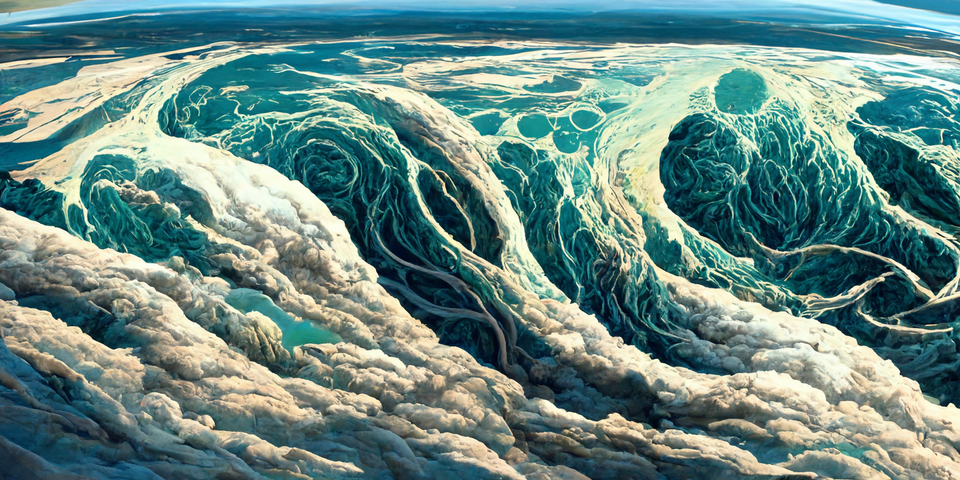
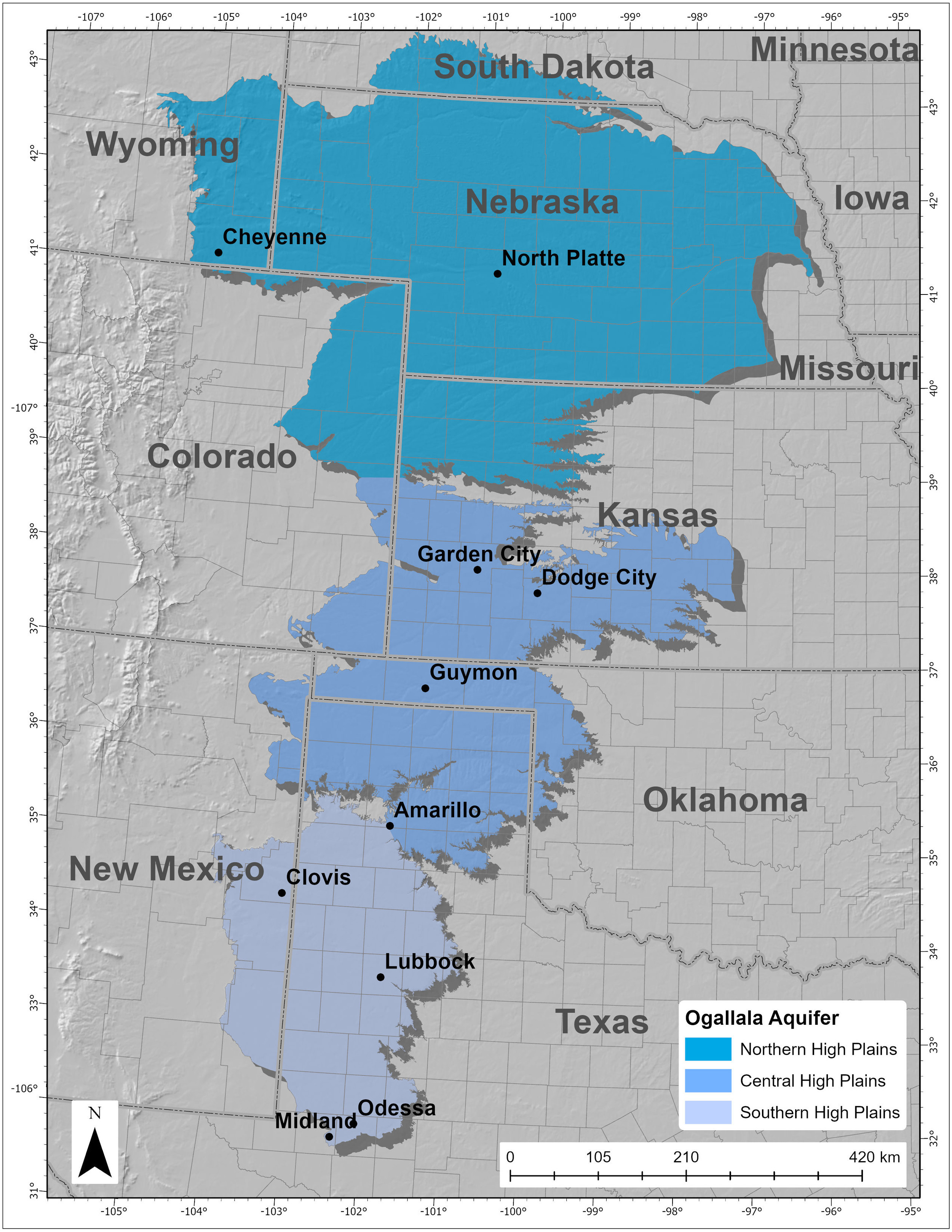
Lubbock, Texas, the Hub City of the South Plains, sits atop the southern range of the Ogallala Aquifer. Thanks to this massive natural formation, in this part of the world, cotton is king because center-pivot irrigation lapping up the fossil water of the Ogallala Aquifer has made these sunny grasslands highly productive. Inside Lubbock’s agricultural history museum, the FiberMax Center for Discovery, a central room of interactive displays depicts scenes from ancient times to the present illustrating humanity’s extraction, conveyance, and conservation of water. Against the background of a center-pivot well watering a lush green field, one panel in particular offers the following statement: “Earth’s water is never really lost. It merely changes location, form or quality.”
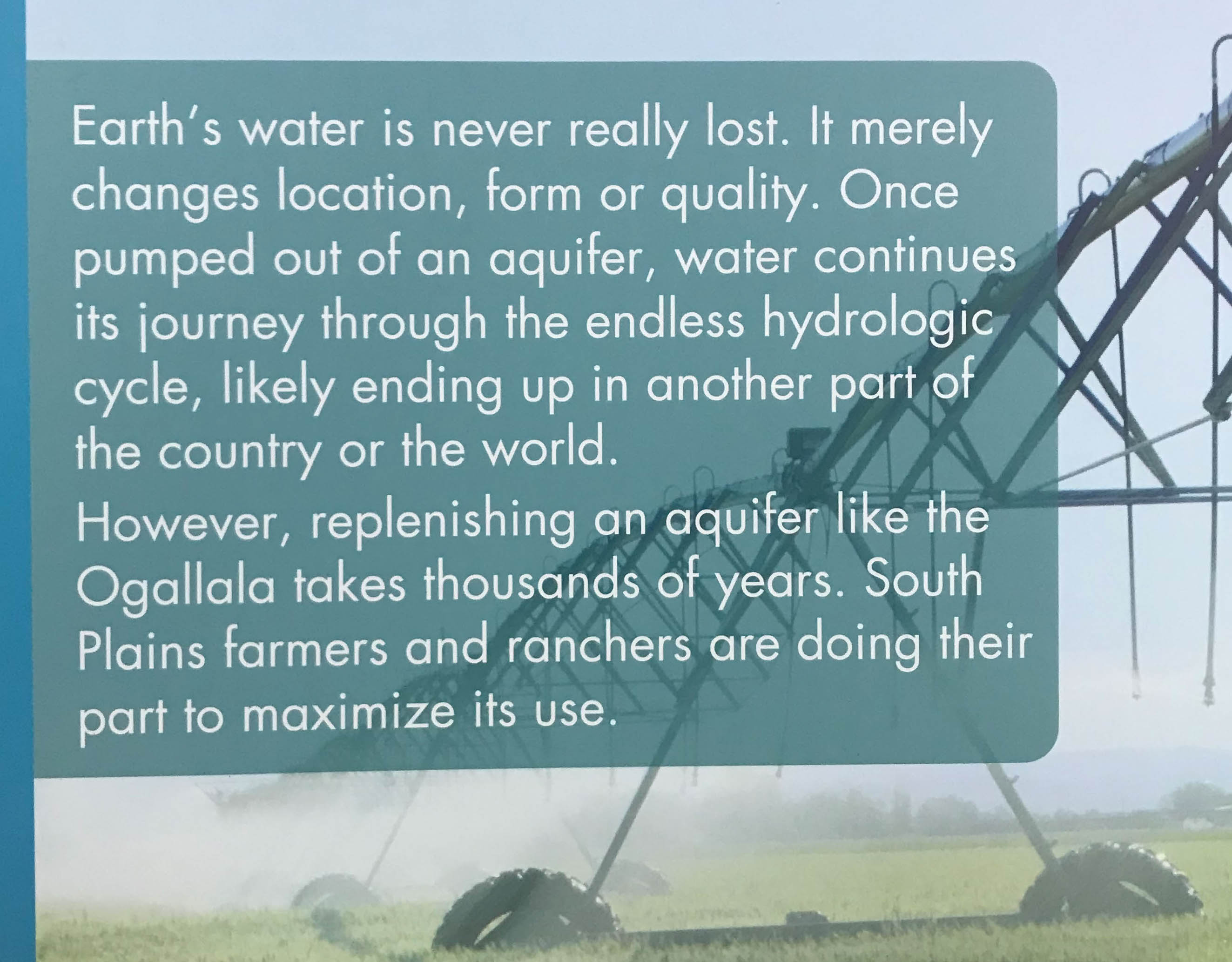
Despite some qualifications, we are encouraged to think that if the water where one currently happens to be ceases to flow, it will always be on hand in some form elsewhere on the planet. Folks on the Llano Estacado are justly concerned about the sustainability of an already significantly depleted Ogallala Aquifer.[1] And in a wider world undergoing climate change and broadly apprehensive about water resources altogether, the FiberMax Center’s reassuring declaration asserts a kind of ultimate guarantee regarding the stability of this priceless substance.
This claim about the absolute conservation of water is presented in a positive spirit of educational enrichment. But one may still ask: is this statement actually correct? Research in Earth system science and astrobiology has raised some important considerations that challenge its unqualified veracity. Indeed, I would suggest that the Center’s contention about unabated water has been mapped onto a much more watertight scientific statement—the first law of thermodynamics, also known as the law of the conservation of energy, the physical axiom stating that energy “is never really lost”—that is, energy cannot be created or destroyed, it can only be converted from one form of energy into another. But even with all its transformative capacities, water is not energy. Composed of two atoms of hydrogen for every atom of oxygen, water is a fundamental molecular form of matter. So the question regarding water’s absolute conservation is a separate issue, one more properly submitted to the laws of chemistry. This article tenders that the availability of water on Earth must also be submitted to the contingencies of biology and geology.
Regarding the science of life, we can certainly honor the depths of wisdom conveyed in the revered saying water is life, while also acknowledging that this semantic equation is not literal but poetic or mythic. Its force comes from figuration, not denotation. Just as the qualities of water cannot be equated with those of energy, so too, literally considered, water is not life. An utterly captivating mode of matter, yes, but it is not itself a living substance. What it is, then, in a secular view, is an indispensable material condition and chemical medium for a planetary environment in which our kind of life is possible. Its gaseous form rises in the clouds that swirl through the sky. Its solid form rests as ancient glaciers, ice cubes in cocktail glasses, and ephemeral snowpacks. Its liquid phase flows over, under, sideways, and down Earth’s rocky surfaces, wending its way through soils and crusts and living things along innumerable paths. Species, kinds, peoples flock where watersheds lead and languish if the water dries up.
Water is a gift, to be sure, nearly as precious as life itself. All the more reason to treat it with due respect and to understand the full terms of its presence in our world. To that end, let us consider the idea that our planet itself may be thought of as a cosmic aquifer—a place that shelters and sequesters water, protecting it from loss, keeping it ready to hand—and in this instance, a place that is an entire planet. Indeed, as NASA, the explorer of other worlds, has ironically affirmed, so far as we know, planet Earth is our only oasis in space.
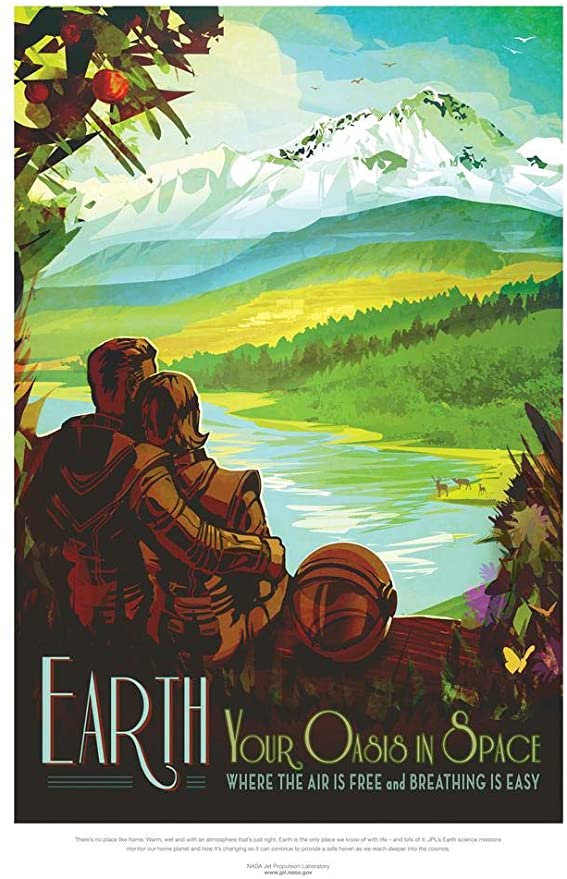
However, just like the Ogallala Aquifer, our planetary aquifer is not an entirely sure or forever thing. Good arguments can be made that the better part of the water ever held on Earth is still sequestered, here on this planet, thanks to Gaia’s—or, if you prefer, the Earth system’s—active role in keeping it here and so keeping the planet fit for life. In other words, planetary water can indeed be lost. But here on Earth, the dynamics of life tied together with its environmental surround have actively retained an enduring if finite abundance of water.
Gaia theorists Stephan Harding and Lynn Margulis make the case for this contention in their 2010 article “Water Gaia: 3.5 Thousand Million Years of Wetness on Planet Earth”:
We suggest that without life’s involvement in complex geological, atmospheric, and metabolic processes, Earth would long ago have lost its water, becoming a dry and barren world much like Mars and Venus.[2]
In the work of Gaia’s primary scientific expositors, James Lovelock and Lynn Margulis, Gaia is defined as the planetary system that, in the final instance, comprehends and coordinates the manifold operational couplings of Earth’s biological and geological systems that produce habitable conditions for the current biota.[3] Developing the implications of Gaian science, Harding and Margulis propose that since attaining its Gaian form—its fully planetary systematicity—life in the aggregate has actively participated with a suite of geological processes to retain Earth’s aqueous endowment, to maintain the surfaces of this planet continuously wet or moist and precisely as such, fit for life. Our water is still here on Earth, this thesis runs, because Gaia has kept it here; and because water and life have persisted, so too has the Gaian system. So it all goes around in a virtuous cycle. This recursive formulation of the Water Gaia thesis models the self-sustaining geobiological cycles that constitute Gaia’s basic operations: in this instance, liquid water makes life possible, so life works out ways to retain the water within the Gaian system, keeping oceans of this priceless molecule available over geological time for its own purposes of self-maintenance and differential regeneration, or evolution.

For contrast—Venus and Mars. Planetary scientists are largely agreed that as young planets, along with Earth, both Venus and Mars had relatively abundant water. It likely came to all three planets from the so-called Heavy Bombardments by water-bearing asteroids that punctuated the final accretion of the rocky planets out of the solar disk some four billion years ago.[4] While Mercury has probably always been too close to the Sun for life to gain a temperate hold, Venus, Earth, and Mars seem to have had comparable endowments of pre-life chemistry and opportunities for vital origins. Moreover, the young sun was fainter, perhaps 30% less luminous than now. For most of its tenure, then, Venus has been within the boundaries of our solar system’s habitable zone, as it’s called, and for life to once have emerged there seems modestly plausible. However, to remain friendly to life over cosmological time, Venus would have needed to achieve a regime of active planetary cooling. It could possibly have sustained a marginally temperate state by establishing a high albedo, or index of reflectivity, bouncing solar energy back into space before it heated the surface, while minimizing the greenhouse gases that hold heat in. And at the other end of the habitable zone, in order to incubate and sustain nascent life, Mars would have needed the opposite—active heating. For this, early Mars might have done the opposite of Venus—work up a strong greenhouse atmosphere to retain surface heat and hold its albedo low so that it converted as much of its sunlight as possible into planetary warming.
Plausible scenarios can thus be constructed for the origin and development of life on both Venus and Mars. However, if life ever got going on either of these worlds, it now seems to be gone. Moreover, and perhaps as a result, both of our neighboring planets are now desiccated in the extreme. The Water Gaia thesis suggests that a major factor in both planets’ drift toward their present arid state has been either the sheer absence of life altogether or the unhappy loss of the nascent life needed to set up the negative or regulatory Gaian feedbacks within their atmospheric regimes that sustain habitable climates for the long haul.
A likely scenario for the cosmic evolution of Venus is the emergence of an intense atmospheric greenhouse that fell into a positive feedback regime of runaway heating: over hundreds of millions of years Venus’s water simply cooked itself away like a stew left too long on the burner, boiling with enough force to expel the bulk of its water out of that planetary system. The smaller planet Mars would have had different problems: its significantly lower gravity was less able to counteract hydrogen loss to space produced by photodissociation of water molecules in its upper atmosphere. As the hydrogen faded away, the leftover oxygen oxidized elsewhere, as we can see in the rusty reddish hue that shows through its nearly cloudless skies. In either case, life was either never present to be a factor or was itself a victim of abiotic cosmological constraints, dying out before any potential system of Gaian type could coalesce to maintain anti-entropic counterforces to the unutterable dryness of space.
Life is precious, for one, because by all current indications it is astronomically rare, a cosmic diamond in the infinite rough, and for another reason, because its very survival and subsequent proliferation appear to depend on a fortuitously rapid burst of initial growth yielding conducive and permanent planetary modifications. A standard explanation for the rarity or absence of robustly evolved life elsewhere in the universe is what astrobiologists Aditya Chopra and Charles H. Lineweaver term the “emergence bottleneck,” that is, the abysmal difficulties of getting life started in the first place. Even in the so-called “habitable zone,” as determined by strictly physical criteria of solar luminosity and distance between star and planet, the complexities involved in getting a starter living system to arise out of a prebiotic mix of molecules and energies are certainly massive. Yet given the age and unfathomable immensity just of the observed universe and the now verified cosmic profusion of exoplanets, one imagines that by now there could have been billions and billions of trial runs for the origin of life. And we do know that, lucky for us, at least one of them succeeded.
Chopra and Lineweaver also offer a supplementary, biological reason for the current state of non-evidence regarding life beyond Earth—the “Gaian bottleneck”:
If life emerges on a planet, it only rarely evolves quickly enough to regulate greenhouse gases and albedo, thereby maintaining surface temperatures compatible with liquid water and habitability. Such a Gaian bottleneck suggests that (i) extinction is the cosmic default for most life that has ever emerged on the surfaces of wet rocky planets in the Universe and (ii) rocky planets need to be inhabited to remain habitable. In the Gaian bottleneck model, the maintenance of planetary habitability is a property more associated with an unusually rapid evolution of biological regulation of surface volatiles than with the luminosity and distance to the host star.[5]
As applied to Venus and Mars, a Gaian bottleneck would imply that, even granting life managed to emerge there, nonetheless, it failed to take control of either planet and so died in the cradle. Or again, it was not able to achieve a Venusian or a Martian version of Gaia and so clinch its own maintenance of habitability. As we have already noted, these living dynamics would have had to involve the persistent retention of water in the liquid phase amenable to all but the most extreme metabolisms. In these scenarios, whatever extraterrestrial life may have managed to emerge elsewhere in our own solar system would have suffered the more common cosmic fate—early elimination—a failure to pass through the Gaian bottleneck.
Planets need to be inhabited to remain habitable.
We see that when it comes to cosmological perseverance, it’s not enough just for life to get going. Infant life is the most fragile. Even as our planet evolved geologically as a rocky world, early life’s own effluents and accumulated deposits reworked its conditions, inevitably altering its chemistry and its terrain. Proliferating species of early life had to evolve along particular pathways that furthered their own continuation and that of their neighbors. One crucial strategy was the formation of symbioses. On Earth, mutualistic relations among different living forms, all microbial at the outset, arose to cultivate and exploit the creative multiplicity of autopoietic possibilities. Gaia theory posits that the inherently diversifying dynamics of all forms of life work in the aggregate to enhance their scenes of habitation. What works for them can spill over to their ecological neighbors, and the life that increases the general flourishing of a community niche tends to persist. The concept of Gaia extends this ecological dynamic to the planet as a whole.
As Harding and Margulis explain, another problem a younger Gaia had to overcome is that any “chemical or physical process that liberates hydrogen from water molecules may, in principle, lead to water loss from a planet” (47). Here on Earth, for instance, some 2.5 billion years ago, photosynthesis evolved in cyanobacteria, the prokaryotic (non-nucleated) organisms long known by the misnomer “blue-green algae.” In the 1980s, genetic sequencing confirmed Lynn Margulis’s radical proposal from the 1960s that the chloroplasts responsible for photosynthesis in all plants evolved from the endosymbiotic incorporation of cyanobacteria, at first by protists (such as algae), the first eukaryotic or nucleated cell types to arise from life’s original kingdom, the bacterial microcosm.[6] That is to say, the evolution of cyanobacteria in the Archean eon bequeathed to almost all subsequent photosynthetic life forms an exquisite metabolic process—autotrophy—by which they feed themselves on nothing more than water, air, and sunlight. Photosynthesis grabs solar photons to split hydrogen from its oxygen partner in water molecules and bind it to ambient carbon atoms, thus producing the carbohydrates—sugars and starches—on which they subsist.
Nonetheless, diatomic hydrogen atoms left unbound to heavier molecules are so nearly weightless that Earth’s gravity alone cannot hold them in. How do living systems keep Earth’s hydrogen from gradually seeping out of the biosphere, leading to an inexorable planetary desiccation? First, let us credit living systems, starting with the microbes, with ways and means to detect and recognize water, avenues to keep it handy even while enduring a drought. Harding and Margulis underscore the cognitive issue at hand in reporting that “Life's sensitivity to water quantity and saltiness seems to be the most elemental of all senses.”[7] Born to water at their origin, living cells also locate and sequester the water needed for their own internal operations by producing and maintaining semipermeable membranes with receptors conducting water into their interiors and the chemistry of water toward their basic metabolic processes. Thus, from the water retained by cellular encapsulation all the way to the biosphere keeping the planetary aquifer well charged, life has invented and practiced any number of ways to supply its perpetual thirst.
"Life's sensitivity to water quantity and saltiness seems to be the most elemental of all senses."
As we’ve noted, the primary producers among the biota that do the oxygenic form of photosynthesis literally take on substance by splitting the hydrogen from water molecules to build carbohydrates. Moreover, all the while, they recycle an environment that continuously reinstates its watery medium. For instance, with a characteristic combination of biological and geological dynamics, one major Gaian cycle starts with the kind of photosynthesis that splits water, binds its hydrogen to carbon, and frees its oxygen as a byproduct:
CO2 + H2O --> CH2O + O2
Yet the oxygen freed biologically by photosynthesis also becomes available to bind once more with and mop up ambient hydrogen molecules. This occurs particularly in the upper atmosphere, where potentially escaping hydrogen tends to congregate. The availability of atmospheric oxygen then effectively brings about the reconstitution and recapture of water through abiological processes of geological mingling and chemical bonding:
2H2 + O2 --> 2H2O
In short, at first a challenge to the biosphere of that moment, the evolution and proliferation of photosynthetic organisms have ensured planetary water retention. This chemical sequence—splitting and reconstituting water in a continuous cycle—is one of a dozen other modes of water retention through biotic and abiotic processes surveyed by Harding and Margulis, from aerobic and anaerobic bacterial processes that sequester hydrogen to the long-term carbon cycle that Gaia theory sees as a primary temperature regulator maintaining the clement conditions that keep liquid water moving through its many-textured pathways.
Finally, in contemplating the Water Gaia thesis, it is important to bear in mind that the Gaian system involves more than just life in the aggregate. Rather, Gaia arises from the mutual interpenetration of living systems, nonliving matter, and energy fluxes from the sun overhead and the deep heat emanating from the Earth’s core. And the most unexpected but telling component of the Water Gaia thesis may well be its sketch for the plausibly mutual coupling of Gaian water retention and the maintenance of the terrestrial system of plate tectonics.
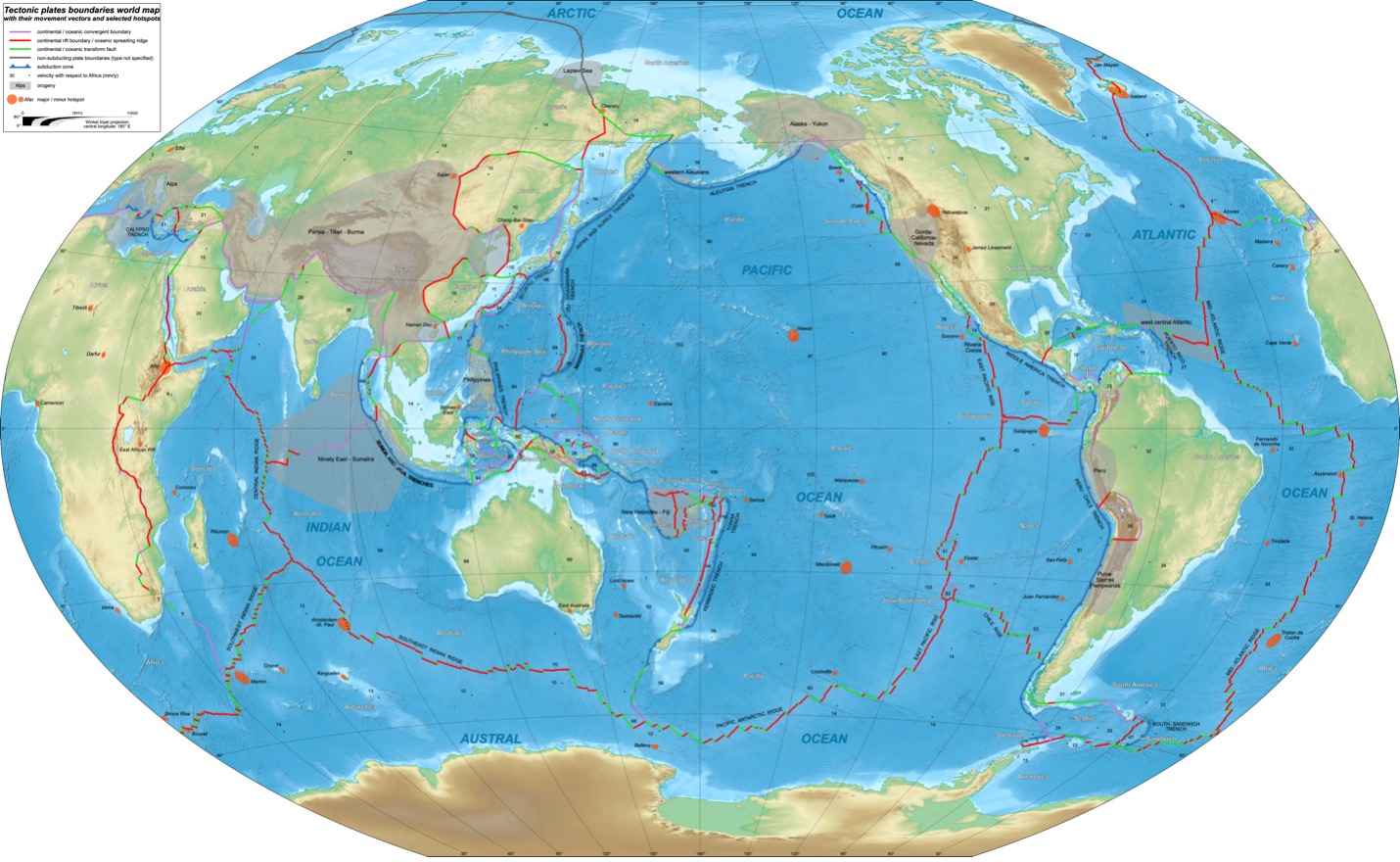
The fault lines that define the plate boundaries primarily occur running down the middle ridges and at the edges of oceans, where the drifting continents well up from the watery seas. Plate tectonics only became a broadly accepted geological super-theory during the same period of the later 20th century in which the Gaia concept also made its appearance. The Gaian view of plate tectonics clinches the message that diversifying life has always been deeply integrated with a co-evolving geological environment. The Water Gaia thesis absorbs the seemingly abiotic dynamics of plate tectonics into a fully Gaian description.
In a recent memoir of his collaboration with Margulis, Stephan Harding summarizes these arguments in “Water Gaia.” “Most profoundly,” he writes regarding their investigations,
we concluded that without life’s water-retaining abilities, there would be no plate tectonics. In essence, water must be present to “soften” sea floor basalt as it plunges downwards at plate margins to melt and be recycled in Earth’s depths. Without plate tectonics there would be no granite and hence no continents, and noreturn of carbon dioxide to the atmosphere and thus no regulation of planetary temperature favorable to the persistence of liquid water and hence of life itself. These massive planetary cycles exist only because the biosphere persistently bonds fleeing hydrogen molecules to those of oxygen, replenishing Earth’s deep wells of water. What “Water Gaia” means for our scientific understanding is that, for the planet as a whole, life itself holds on to the water it needs to live and flourish.[8]
The Gaian mechanism here is precisely the continuously replenished presence of oceans of water moving between the biosphere and the geosphere. According to this corollary of the Water Gaia thesis, because Gaian processes actively and continuously prevent the loss of hydrogen from Earth’s precincts and its recapture for the biosphere, the Gaian system ensures the terrestrial presence of sufficient water to carry out the various forms of mineral hydration and lubrication necessary to the keep the dynamics of plate tectonics in motion. In turn, plate tectonics ensure the continuous redelivery of subducted carbon, particularly by way of volcanism, back to the atmosphere as a feedstock for continued photosynthesis. Harding and Margulis suggest that these precise considerations yield “an interesting and appropriately circular Gaian dynamic . . . : no life, no water --> no water, no plate tectonics --> no plate tectonics, no life.”[9]
To sum up, then, Earth’s hydrological cycles are vastly more integrated into and dependent upon the totality of the biosphere and the maintenance of its conditions of habitability than we had previously thought. Water does not just blithely go around in circles as it runs its planetary courses. Rather, in a manner parallel to life itself, because it is continuously broken down and reassembled by life’s own primary processes, over geological time water is continuously subjected to the threat of cosmic dissipation. But instead, in a virtuous circle as big as the planet, it sustains the life processes that prevent its loss from the Earth system. The geological dynamics in play fall into place within the Gaian system. Water’s global movements also run around and through the organic pulsations of living systems. Its flows are processed both through biological pumps—for instance, by evapotranspiration in plants—and by passage through its rocky channels, whose biotically-enhanced weathering by water flow releases nutrients locked up in geological formations from previous eons.
The Water Gaia thesis is an especially powerful reminder that no Earthly formation can be taken for granted either as timeless and eternal or as independent of far wider environmental and ecological contingencies. The active protection of what we humans, in our species-centered way, like to call “natural resources” would seem to be a thing that Gaia has been doing on its own behalf for the three billion years of its operation. Viewing this regional formation against its planetary horizon, we can see the Ogallala Aquifer as one among many tributaries of an Earth system whose replenishment is inextricably tied to Gaia’s own persistence.
The initial version of this article was delivered on April, 2022, at the 54th Comparative Literature Symposium at Texas Tech University, Perspectives on Water on the Llano Estacado, directed by John Beusterien. Thanks to John for his encouragement, and Sankar Chatterjee, Tyler Volk, and David Schwartzman for helpful critiques.
References
Chatterjee, Sankar. From Stardust to First Cells: The Origin and Evolution of Early Life. Springer Verlag, 2023.
Chopra, Aditya, and Charles H. Lineweaver. “The Case for a Gaian Bottleneck: The Biology of Habitability.” Astrobiology 16:1 (2016): 7–22.
Clarke, Bruce. Gaian Systems: Lynn Margulis, Neocybernetics, and the End of the Anthropocene. Minneapolis: University of Minnesota Press, 2020.
Clarke, Bruce, and Sébastien Dutreuil, eds. Writing Gaia: The Scientific Correspondence of James Lovelock and Lynn Margulis. Cambridge: Cambridge University Press, 2022.
Harding, Stephan. “Gaia and the Water of Life.” In Clarke and Dutreuil, Writing Gaia, 400-403.
Harding, Stephan, and Lynn Margulis. “Water Gaia: 3.5 Thousand Million Years of Wetness on Planet Earth.” In Eileen Crist and Bruce H. Rinker, eds. Gaia in Turmoil: Climate Change, Biodepletion, and Earth Ethics in an Age of Crisis. Cambridge, MA: MIT Press, 2010. 41–59.
Little, Jane Braxton. “Saving the Ogallala Aquifer.” Scientific American Special Editions 19 (March 2009): 32-39. doi:10.1038/scientificamericanearth0309-32.
Margulis, Lynn, and Dorion Sagan. What is Life? Berkeley: University of California Press, 2000.
van Thienen, Peter, Karim Benzerara, Doris Breuer, Cedric Gillmann, Stephane Labrosse, Philippe Lognonné, and Tilman Spohn. “Water, Life, and Planetary Geodynamical Evolution.” Space Science Reviews 129 (2007): 167-203.
[1] See Braxton, “Saving the Ogallala Aquifer.”
[2] Harding and Margulis, “Water Gaia,” 41. A related approach to this overall topic is van Thienen et al, “Water, Life, and Planetary Geodynamical Evolution.”
[3] See Clarke, Gaian Systems.
[4] See Chatterjee, From Stardust to First Cells.
[5] Chopra and Lineweaver, “Gaian Bottleneck,” 7.
[6] See Margulis and Sagan, What is Life?, 103-6.
[7] Harding and Margulis, “Water Gaia,”45.
[8] Harding, “Gaia and the Water of Life,” 402-3. See also the section “Water Gaia” in Clarke and Dutreuil, Writing Gaia, 320-21.
[9] Harding and Margulis, “Water Gaia,” 55.
Member discussion